More than 4 decades of kaon experiments in CERN's North Area proved how a small number changed the universe.
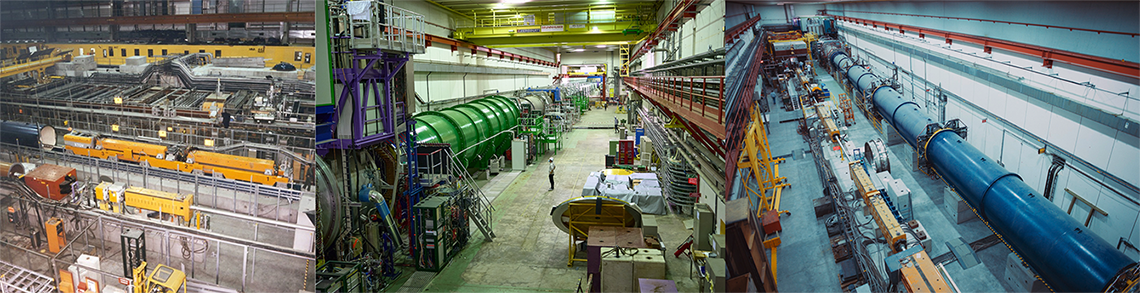
The recent history of kaon physics experiments at the SPS started in the beginning of 1980 in the office of the later Nobel Prize winner Jack Steinberger with a small team of physicists including Heiner Wahl, Don Cundy, Italo Mannelli, and Niels Doble. They discussed the design of the NA31 experiment that aimed to discover the existence of the so-called Direct CP Violation. CP is the product of the operators C and P, where C replaces all charge quantum numbers (electric charge, strangeness, etc.) by their opposite and P inverts all space coordinates (i.e. looking in a mirror). The combination CP of these two operators was believed to conserve symmetry, but 60 years ago to the day, in 1964, Cronin, Fitch, Christenson, and Turlay published that CP was not conserved in the weak interaction leading to the Nobel prize in 1980 for Cronin, Fitch, and Christenson.
The neutral strange particles directly produced in the strong interaction are K0 and K0. A symmetric and anti-symmetric mixing of K0 and K0 explains their different lifetimes and are referred to as KS and KL. If CP is conserved, the short-lived neutral kaon, called KS, must always decay into two pions and the 600 times longer lived KL into three or more particles. But Cronin et al discovered that in a few permille of the cases KL also decays into two pions. The different decay channels and lifetimes of KS and KL were initially explained by perfectly symmetric and anti-symmetric mixing of K0 and K0 themselves.
Cronin et al observed evidence for CP Violation, which was attributed to a deviation from perfect symmetry and asymmetry in the mixing. Later theory predicted that in that case there could also be a difference in the decay rates of K0 and K0 themselves, i.e. a difference between the decay rates of particles and anti-particles. This so-called Direct CP violation could therefore contribute to the matter-anti-matter asymmetry in the universe (knowing of course that ordinary matter is not made of kaons but of protons, neutrons and electrons).
In the neutral kaon system, Direct CP violation manifests itself by a difference from 1 of the double ratio of KL decaying into either two charged or two neutral pions and KS decaying into the same final states, which is called the R ratio and defined by John Ellis, Mary Gaillard, and Dimitri Nanopoulos. Theory predicts R to be equal to 1 – 6ε’/ε, where ε is the parameter describing CP violation through mixing and ε’ the one describing Direct CP violation; ε is as small as 2.228 × 10-3. This small difference changed the universe. The experiments obviously need both a KL beam and a KS beam and the detectors must count the decay rates into two pions with extreme precision.
In KS, almost all decays are into two pions and there is therefore almost no background from other modes, but the KL decays are mostly (more than 99.7%) into other decay modes that may mimic two-pion decays, e.g. by missing a decay particle. The experiments need relatively high beam intensities to collect sufficient statistics and therefore particles produced by interactions of different protons in the target may sometimes look like decay products of a single kaon. This pile-up is one of the major systematic backgrounds in all kaon experiments. Obviously, the detectors and the beam lines must work together in perfect coordination, hence the very close involvement of beam experts in these experiments. Another complication is that at the typical kaon energies used (about 100 GeV) the average KS decay length is about 6 m and the average KL decay length 3 km. Therefore, the longitudinal distribution of the decay points (vertices) is very different for KS and KL.
NA31 solved the latter problem by putting the KS target together with two magnets on a train. The train ‘served’ 41 station positions separated by 1.2 metres. As the train took about a minute for the trip from one station to the next, it was called XTGV. The resulting KS decay vertex distribution was a saw-tooth distribution close enough to the almost flat KL decay distribution. This, together with the decision not to use a spectrometer magnet for the charged decays, essentially eliminated the need of Monte Carlo simulations. The experiment design was very elegant and largely driven by the beam design. A technical complication was the fact that the train had to run inside the big blue vacuum tank. Inside vacuum the conductivity of air has a maximum around 10-1 mbar and this complicates the functioning of the magnets and the photomultiplier based scintillator filament scanner (FISC) before the target.
Therefore, a good vacuum was required in spite of the outgassing of the magnets. The experiment, including the 100 m long blue vacuum tank, was located in the H4 beamline in EHN1. The experiment was a success and published the first observation of Direct CP violation with 3σ precision (still considered sufficient at that time): ε’/ε = (2.0 ± 0.7) × 10-3. Unfortunately, the E731 experiment in Fermilab found a result consistent with zero and both experiments decided to go for even more precise measurements. By the way, in that period also 5σ started to become the norm required to claim discovery.
At CERN the next experiment was NA48, which included several lessons learnt from its predecessor NA31. Pileup turned out to be the largest systematic uncertainty in NA31 and therefore the KS and KL beams had this time to operate simultaneously. For the detector there should be no bias between the acceptance for the charged and neutral decays and the beam lines has to be as collinear as possible.
Nevertheless, one had to be able to attribute a given K0 decay to either the KS or the KL beam. The number of events and correspondingly the beam intensity had to be higher to reach 5σ evidence and the blue tube therefore had to move from the H4 beam line into ECN3. The beam design involved one of the most exciting studies we did over all these years; the use of a bent crystal. This crystal was put just downstream of the KL target and recuperated the required small fraction (5 × 10-5) of the non-interacting protons. Those were subsequently transported along the KL beam axis and finally deflected by a dipole magnet onto a KS production target 120 metres downstream and laterally only 72 mm away from the KL beam axis. From there the two neutral beam lines converged gently with a very small angle of 0.6 mrad to the NA48 detectors, which now included the magnetic spectrometer MNP33. A bit downstream of the bent crystal, the protons were tagged by a small scintillating hodoscope and associated with the event in the main detectors via time-of-flight. The different decay vertex distributions were corrected for by lifetime-weighting.
The experiment operated very well and the final result reached a precision of almost 7σ: (14.7 ± 2.2) × 10-4, in nice agreement with the NA31 result and excluding the E731 result. In parallel the new Fermilab experiment, KTEV, got now a result nicely compatible with NA48 and with similar precision. These are still the best measurements of ε’/ε until now. Often people thought the experiment would only publish this one number, which turned out to be completely wrong: NA48 published tens of additional papers on many rare kaon decay channels.
The experiment also developed very innovative detector technologies, the most impressive one being the liquid krypton calorimeter. The calorimeter is a large cryostat filled with about 9000 litres of liquid krypton (about one year of world production) at 120K and a very thin electrode structure with 13,000 channels. At the time this was a very large number of channels for a calorimeter. The design was very innovative. Thanks to be essentially fully active, the performance was excellent, both in energy and time resolution as well as uniformity of response, It still serves today, The KL beam design has served as basis for the HIKE phase 2 beam design, proposed last autumn.
When NA48 concluded, the collaboration wanted to study possible CP violation in charged kaon decays. This was done by comparing the decay distributions (the so-called Dalitz plots) in K+ and K- decays into three charged pions.
Encouraged by the success of the simultaneous beams concept, simultaneous K+ and K- beams were designed and quickly installed by adding an achromat of 4 dipoles following the T10 production target during the 2002-2003 YETS. The first two dipoles provided opposite vertical offsets for K+ and K- at the TAX, which would be compensated by the last two dipoles of the achromat. The TAX had holes that allowed to select either one or both beams. There was only very weak focusing with four weak quadrupoles from the T10 target to experiment that introduced no difference between positive and negative beams. Note that a similar concept is now under consideration for the NUTAG experiment. The detector was the same as for the original NA48 experiment, apart from the addition of innovative small TPC-like trackers, KABES, with minimal material budget. The experiment provided a measurement of unprecedented precision, but no evidence for CP violation was achieved with the desired precision. The last paper of NA48/2 is actually in preparation just now!
During the running of NA48/2, the proposal for NA62 was being prepared and submitted in June 2005. The experiment was approved by the Research Board only at the end of 2008. While waiting, the collaboration used the existing beam (but with K+ only) to measure precisely the ratio between the K+ → e+ν and K+ → μ+ν branching ratios. NA48/3 provided again the most precise measurement so far, in agreement with the Standard Model. At the same time, it was an excellent preparation for NA62, with only one charged track in the final state.
Following the approval of NA62, the construction of the new higher-intensity beam line and of the new experiment started. NA62 aimed to measure the branching ration of the very rare decay K+ → π+νν to 10% precision. The measurement is excessively difficult, as the neutrinos cannot be detected. The SM branching ratio is 8 × 10-11 and this implies that the experiment must be very certain that they have not overlooked any other particles than neutrinos at this level. Indeed, they had to slightly adapt their goal and now expect to reach about 15% by LS3. NA62 is particularly well-known for its flagship measurement K+ → π+νν. However, similarly to NA48, the experiment has published numerous other world-leading papers on many different topics, such as lepton number violation, lepton flavour violation and even dark matter searches.
In parallel to running the beam line for NA62 physics data-taking, the P42 beam serves as a test bed in preparation for the much higher intensity beams expected after LS3. This very important kaon research line has made great contributions to kaon physics and even to particle physics as a whole. It is complementary to CP-violation physics in the B-sector with LHCb and Belle-II and in synergy the combination with their results allows to better refine the knowledge of the Standard Model.
All kaon experiments rely on a close interplay between the detectors and the beam line. Therefore, they always have been a fantastic playground for the BE-EA beam physicists with many difficult challenges and innovative approaches.
But also the technical teams in the group could regularly demonstrate their expertise: A few telling examples are the XTGV train in vacuum in NA31, as well as the thin 2 m diameter Kevlar windows separating the decay volume from a Helium tank at 1 bar for NA31 and NA48, the bent crystal technology and its precise goniometer and the delicate KS collimator for NA48, a new beam dump 24 m underground behind ECN3 and recently the hydrogen Cedar for NA62. But there are too many to all be listed here.
After LS3 the ECN3 cavern will be attributed to the SHiP experiment, an ambitious experiment to explore the dark sector and in parallel to collect large amounts of neutrino interactions, including νt and νt. This marks the end of a very important and successful program of more than 40 years of kaon physics at CERN.